ISOP has received funding from the European Union’s Horizon Europe research and innovation programme, Marie-Sklodowska-Curie Actions (DN-ID), under Grant Agreement No. 101073266. Views and opinions expressed on this website are however those of the author(s) only and do not necessarily reflect those of the European Union. Neither the European Union nor the granting authority can be held responsible for them.
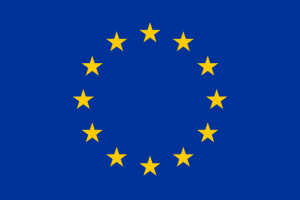
AIM
The AIM of this four-year work programme is to undertake cutting edge multidisciplinary research and development to make a step change in understanding and advancing Supercritical CO2 based power generation systems’ technology. This will enable a step change in the role played by power and heat cycles to become major contributors to achieving the 2050 zero emissions targets. ISOP will achieve this goal while providing specialised training for 17 doctoral researchers to help establish the backbone of an important industry.
Background
The large reliance on fossil fuels to provide heat and electricity through thermodynamic power cycles, which started with the first industrial revolution, has led to adverse impact on human health and climate change and to fast depletion of resources. There has been a rapid growth in the deployment of renewable energy technologies in recent years, such as solar PV and wind, which no longer rely on thermodynamic power cycles. However, to meet the 2050 zero emissions targets, it is widely acknowledged that a broad portfolio of energy technologies will be required. This is likely to include nuclear, biofuels, concentrated-solar power (CSP) and the implementation of technologies to improve efficiency, such as waste-heat recovery, and even the continued use of fossil fuels with carbon capture and storage. Hence, thermodynamic power cycles will remain a pivotal component of the power supply mix. Significant enhancement in the performance and cost reduction of thermal power plants necessitates innovative new approaches.
Modern thermal power generation cycles are required to offer step increase in performance, flexibility, resilience and responsiveness to balancing supply and demand. A promising cycle that could satisfy these characteristics, whilst offering step increase in conversion efficiencies compared to conventional Gas Turbine, Steam Rankine and Organic Rankine Cycle plants, is the Joule-Brayton cycle using supercritical carbon dioxide (sCO₂) as the working fluid.
Favourable properties of CO₂ include a low critical temperature, which enables operation in the supercritical region, high fluid densities that facilitate reduced compression work, very high specific power output as well as significant reduction in the plant footprint. CO₂ is also low cost, non-flammable, and stable at high temperatures.
Despite the substantial potential and increasing interest, sCO₂ systems have not been commercialised yet due to technical challenges in system integration, operation and control, component design material selection and manufacturing technologies. In addition, there are challenges in cycle design required to maximise efficiency whilst reducing system complexity and cost. In particular, high pressures and temperatures, in excess of 200 bar and 450°C, alongside the reactivity of CO₂, require careful material selection and advanced component and system fabrication techniques to ensure safe operation. Whilst high pressures lead to high densities and small physical footprint, they introduce design challenges for the high power density turbomachinery components and heat exchangers. Large variations in the thermodynamic properties of CO₂ close to critical point also introduce significant challenges.
The large reliance on fossil fuels to provide heat and electricity through thermodynamic power cycles, which started with the first industrial revolution, has led to adverse impact on human health and climate change and to fast depletion of resources. There has been a rapid growth in the deployment of renewable energy technologies in recent years, such as solar PV and wind, which no longer rely on thermodynamic power cycles. However, to meet the 2050 zero emissions targets, it is widely acknowledged that a broad portfolio of energy technologies will be required. This is likely to include nuclear, biofuels, concentrated-solar power (CSP) and the implementation of technologies to improve efficiency, such as waste-heat recovery, and even the continued use of fossil fuels with carbon capture and storage. Hence, thermodynamic power cycles will remain a pivotal component of the power supply mix. Significant enhancement in the performance and cost reduction of thermal power plants necessitates innovative new approaches.
Modern thermal power generation cycles are required to offer step increase in performance, flexibility, resilience and responsiveness to balancing supply and demand. A promising cycle that could satisfy these characteristics, whilst offering step increase in conversion efficiencies compared to conventional Gas Turbine, Steam Rankine and Organic Rankine Cycle plants, is the Joule-Brayton cycle using supercritical carbon dioxide (sCO₂) as the working fluid.
Favourable properties of CO₂ include a low critical temperature, which enables operation in the supercritical region, high fluid densities that facilitate reduced compression work, very high specific power output as well as significant reduction in the plant footprint. CO₂ is also low cost, non-flammable, and stable at high temperatures.
Despite the substantial potential and increasing interest, sCO₂ systems have not been commercialised yet due to technical challenges in system integration, operation and control, component design material selection and manufacturing technologies. In addition, there are challenges in cycle design required to maximise efficiency whilst reducing system complexity and cost. In particular, high pressures and temperatures, in excess of 200 bar and 450°C, alongside the reactivity of CO₂, require careful material selection and advanced component and system fabrication techniques to ensure safe operation. Whilst high pressures lead to high densities and small physical footprint, they introduce design challenges for the high power density turbomachinery components and heat exchangers. Large variations in the thermodynamic properties of CO₂ close to critical point also introduce significant challenges.